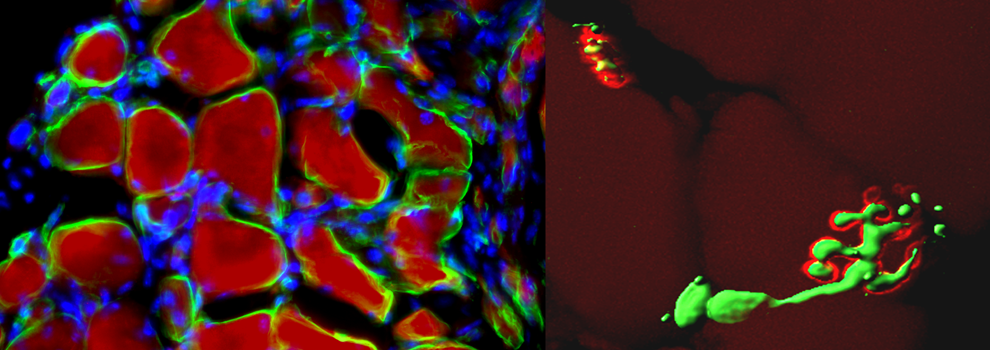
a) Stem cells and regenerative medicine have raised a remarkable interest in the scientific community for their promise to recover aged, injured and diseased tissues. However, despite this noteworthy potential, hurdles currently hinder their clinical application: cell survival, immune response, tissue engraftment and efficient differentiation. In this context, tissue engineering aims to mimic the environment where organogenesis took place and thus allow better stem cell engraftment and consequent new tissue histogenesis. Increasing emphasis has been placed on the features of biomaterials that need to be inert, biocompatible, biofunctional, bioactive and resorbable to ensure optimal (specific) cell-matrix interactions and cell signaling. Enhancing skeletal muscle regeneration and/or reconstruction using tissue engineering would be invaluable for the treatment of a variety of muscle diseases such as localized muscular dystrophies, post-traumatic or post-surgery tissue ablation and reconstruction of incontinent sphincters. To date however, no satisfactory re-building of skeletal muscle has been reported, likely due to the fact that reconstructing this tissue requires parallel alignment of newly formed muscle fibers, each surrounded by a dense capillary network and properly innervated. Scaling up this process for reaching the size of a mammalian muscle of a large animal or a patient creates the problem of angiogenesis that cannot progress in vivo before the occurrence of hypoxia induced. Two general approaches are adopted to engineer skeletal muscle tissue: one is to isolate muscle progenitor cells from biopsies, expand and differentiate cells in a 3D defined environment in vitro (biomaterial or bioreactor) and reimplant the differentiated neotissue (in vitro tissue engineering). The second approach forecasts isolation of muscle stem cells, expansion in vitro and reimplantation of donor cells using a transport matrix, which promote myotube differentiation (myoblast transfer therapy). Moreover, muscle progenitor cells could be modified as vehicle for recombinant protein delivery (in vivo tissue engineering). Our efforts are focused on engineering skeletal muscle tissue by combining vessel associated myogenic progenitor cells (Mesoangioblast, Mabs) with the scaffold component represented by PEG-Fibrinogen (PF), an injectable hydrogel. Our research project follows two main directions: in vivo myoblast transfer therapy for skeletal muscle tissue regeneration and in vitro tissue engineering for muscle reconstruction.
2D Mabs plastic culture shows myotube differentiation within 3-5 days after serum depletion, while using PF as scaffold for 3D Mabs culture, myofiber formation occurs in 24 hours, revealing the capacity of PF to promote and accelerate muscle differentiation in vitro. Moreover in vivo experiments conducted in a muscle injury mouse model (cardiotoxin crushed) using PF as carrier for Mabs injection demonstrated increased cell survival, ameliorated engraftment of transplanted cells likely due to improved integration and fusion of injected cells with host regenerating muscle fibers.
As for muscle reconstruction in vivo, we implanted PF and n-LacZ expressing Mabs, modified to express the angiogenic factor Placental derived Growth Factor (Mabs-nLacZ/PlGF) subcutaneously into immunocompromised mice. We are attempting to implant PF loaded with Mabs-nLacZ/PlGF subcutaneously on the surface of the Tibialis Anterior of immunocompromised mice in order to investigate whether natural mouse TA movement could lead to parallel orientation and hence functionality of the artificial muscle.
Preliminary data demonstrated that TA contraction movements influence fiber organization and alignment in the muscle-like tissue while implant survival is assured by host blood supply recruited by PlGF action, thus opening the possibility by PF and Mabs tissue engineering to develop a complete artificial muscle patterning in different shape and size. Thus the future work is to elaborate this approach in a translational biotechnology in order to reconstruct segment of muscular tissue and graft than to substitute whole damaged or failing muscles; moreover since this structure is able to be modulated in elasticity, resistance and shape depending on application, could have a remarkable role on different muscle tissue reconstruction: groin hernia, traumatized or oncological removal treated muscle and muscle replacement for sphincter incontinence. Therefore the next approach would be focus on tissue-engineer a muscle in a bigger mammal in order to reproduce a complete and functional piece of artificial muscle and grafting it to replace an injured, diseased or failing muscle.
b) Muscular dystrophies (MD’s) comprise a heterogeneous group of diseases characterized by primary muscle fibers wasting and progressive loss of strength, that in severe case likewise Duchene Muscular Dystrophy (DMD) leads to wheelchair dependence, respiratory failure and premature death (Emery 2002). In MD’s lacking or mutating on dystrophin or dystrophin-glycoprotein related members, protein complex linking muscle contraction machinery to the basal lamina, drives to muscle fragility and consequent myofibers degeneration accompanied by a severe inflammation with infiltration of immune cells. Inflammation has long been recognized as feature of MD’s but just as side phenomenon reflecting non-specific inflammatory response to muscle damages. Degenerating fibers are repaired and replaced by myogenic resident stem cells (satellite cells), nevertheless dystrophic satellite cells produce muscle fibers prone to degeneration as well. Thus after several cycle of damage and repair, when they exhausted their regenerative capability, degenerating fibers are progressively substituted by connective and fatty tissue leading to a sclerotic environment with a remarkable microcirculation reduction and a severe chronic inflammation. This is the case of muscular dystrophy at advanced stages of the disease, representing the vast majority of affected individuals. Although molecular defect and ongoing mechanism of the disease are known, satisfactory therapy is not available, being still far from yielding complete skeletal muscle recovery. For pharmacological, gene and cell therapy, efficient delivery of the therapeutic drug, gene or cell to skeletal muscle represents the major challenge since skeletal muscle is the most abundant tissue in the body and is composed of multinucleated cells that do not divide. Furthermore, the pathological changes that occur in the advanced stages of the disease will reduce the efficacy of molecules, gene and cell delivery: mesoangioblasts (Mabs), vessel associated progenitor cells, treatment was greatly reduced when it was administered to mice older than two months of age. Even small molecules such oligonucleotides or drugs may loose efficacy when a dramatic reduction of the microcirculation may prevent their reaching the surviving muscle fibers. Therefore, the current trials that may be successful on young patients they would be unlikely to release a significant benefit when extended to cases at more advanced stages of the disease, the vast majority of affected individuals. We have recently demonstrated that tendon fibroblasts engineered to express a metalloproteinase and an angiogenic factor can restore microcirculation and reduce connective tissue deposition after intramuscular delivery to old dystrophic muscles. This treatment causes a dramatic amelioration of successive intra-arterial cell therapy that is restored to the same level of efficacy observed in young dystrophic muscle.
Hence in the light of above-mentioned results we are developing a new modular technology enabling a complete, groundbreaking regeneration of skeletal muscle. This technology consists of the combination of new generation of fibrinogen biomaterial, the PEG-Fibrinogen, and mesoangioblasts. The field of regenerative medicine as a whole has faced two main obstacles: the first one is the difficulty, when using stem cells, of localizing the therapeutic effect to the target tissue in a specific manner. Previous clinical attempts of muscle regeneration in humans involving the resident myogenic precursors located underneath the muscle fiber basal lamina or satellite cells have failed partly because of their limited migratory capacity and survival. They tend to remain at the injection site vicinity, making it difficult to achieve an even distribution throughout the muscle and to go trough programmed cell death. Another reason for the failure of previous cell therapies is the fact that stem cells have not been protected adequately. The combination therapy we are attempting has not only proven to address these two obstacles when tested for its regeneration capacity in DMD animal models, but also showed superiority in the degree of regeneration obtained when compared to the mesoangioblasts alone.
c) Limb regeneration is one of the best examples of regeneration in vertebrates, since limb is a complex structure composed of different tissues. Urodele amphibians (newts and salamanders) are able to regenerate amputated limbs anytime during their life, while anuran amphibians (frogs) can regenerate their limbs during larval stages but progressively lose this capability during development until metamorphosis occurs. Mammals do not regenerate amputated limbs during adulthood but they possess the ability to regenerate digit tips during pre-natal and neonatal life. Limb regeneration in urodeles is due to the formation of an apical epithelial cap (AEC), which then induces the formation of a regenerative blastema. The blastema consists of a population of active proliferating progenitor cells and arises from dedifferentiation of stump tissues cells. It contains the intrinsic morphogenetic informations that are required to re-pattern the regenerating limb and direct the regenerative response. In spite of the deep knowledge on molecular mechanism occurring during amphibian limb regeneration processes the cellular movement, fate and commitment need to be elucidated yet. Since the idea is to focus on study Xenopus laevis limb blastema cells identity. Recently we succeeded in elaborating a protocol for limb blastema stem cells isolation, growth and labelling. Limb blastema at different regeneration stages will be treated in order to obtain isolated stem cells; to understand their potentiality, freshly isolated blastema stem cells will be labelled (Lentiviral vector), implanted in regenerating and non regenerating amputated tadpole limb to analyse their capability in promote regeneration of all missing appendage or limited to specific tissue or structure related to their stage of origin and than to their differentiative state.
Mammals appendage regeneration is extremely reduced, during digit tip amputation in mice, a typical inflammatory response occurs: neutrophils and monocytes enter the damaged site, differentiate into macrophages and attract fibroblasts. Fibroblasts begin synthesizing collagen and extracellular matrix proteins, which turn into a scar tissue. It hampers the intercellular signalling between the epidermis and the deeper tissues of the wound, avoiding the formation of an AEC and a blastema. That is one of the principle reasons why limb regeneration is impaired in adult mammals; in contrast, wounds in embryonic and foetal mammals heal without scar formation and involve a smaller inflammatory response, so that a partial regenerative response is possible. A “conservative” treatment in clinical cases of digit tip amputation in humans, by delaying the wound closure, in fact seems to promote a regenerative response. Since the idea to promote mouse digit tip regeneration pursuing three different approaches:
- cell therapy based on GFP labelled mesoangioblasts, vessel-associated progenitor cells, genetically modified for the expression of metalloproteinase-9 (Mabs-MMP9) in order to avoid scar tissue deposition and promote tissue regeneration;
- gene therapy based on third-generation lentiviral vectors to promote Dominant positive beta-Catenin (Dpbcat) expression which encodes for a dominant positive form of b-catenin in order to up regulate the Wnt/b-catenin pathway, it has been already demonstrated its necessary role during limb regeneration in amphibians by inducing blastema cell proliferation;
- biomaterial use (PEG-fibrinogen, PF) in order to allow a gradual and controlled viral vector release in vivo or to support the delivery of modified mesoangioblasts into the amputated digit tip.
The treatment of amputated digits of 14-day-old mice with Mabs-GFP/MMP9 results on formation of a loose cell layer whose morphology reminds an early blastema. This cellular cap could be due to the proliferation of the injected mesoangioblasts or it could appear after the mobilization of local progenitor cells or after the proliferation of dedifferentiated cells. Immunohistochemical analysis revealed that the injected mesoangioblast did not take part to the observed cellular cap in the treated mice. Considering that MMP9 is a secreted protein able to spread throughout the microenvironment, it is likely to think that the formation of the loose cellular cap in the treated mice was due to the action of MMP9 molecules rather than to the proliferation of mesoangioblasts. The secreted MMP9 molecules could degrade the network of extracellular matrix, which is deposed by fibroblasts during the inflammatory response following amputation, and so they could permit the movements of cells and the diffusion of soluble signalling molecules.
In a similar way to the events of limb regeneration in amphibians, the up-regulation of Wnt/b-catenin signalling pathway in the amputated digit tips of newborn mice leads to an increased proliferation, PF has been used in association with third-generation recombinant lentiviral vectors for the expression of Dpbcat in order to promote the same effect of Wnt/b-catenin pathway: the formation of a regenerative blastema or a blastema-like structure. Lentivirus-based gene therapy could be more efficient in in vivo applications if the lentiviral vectors were able to infect target cells as long as possible. The association of lentiviral vectors with biomaterials could increase their efficiency by protecting them from the surrounding environment and from the host immune response and also by slow releasing in loco. In vitro, PEG-fibrinogen was able to preserve the stability of recombinant lentiviral vectors for the expression of a reporter gene and it was able to release them gradually, because of biomaterial properties of biodegradability and mesh. All these data strongly support the efficacy of the novel approach using biomaterial to promote limb regeneration in vertebrates. The use of biomaterials, like PEG-fibrinogen, in regenerative medicine could be promising because they are specifically designed to be degradable in vivo during the physiological processes of tissue remodelling and so they could behave like a scaffold for modified mesoangioblasts in cell-based therapies or for third-generation recombinant lentiviral vectors in gene therapy approaches.
Università di Tor Vergata